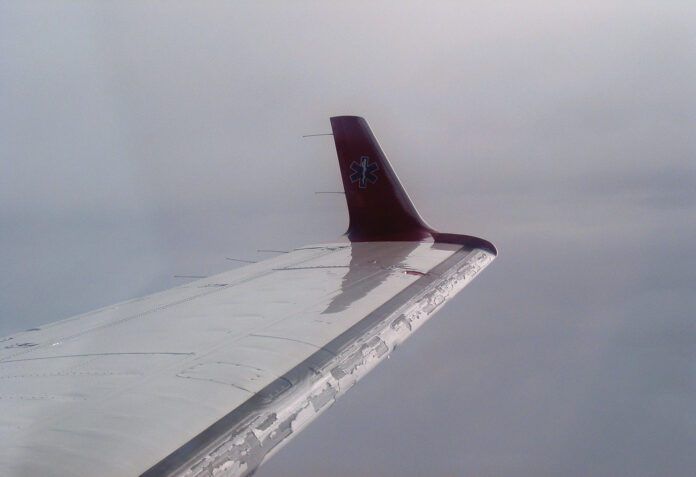
Some subjects in aviation are covered during flight training in an academic sense, but their practical application presents challenges. For example, most of what I was taught about SIDs and STARs involved writing, “No SID/STAR” in the flight plan form’s remarks section. In a similar vein, most of what I learned about in-flight icing while bombing around in a Skyhawk was avoidance: Recognize the symptoms and find the fastest way out.
Once I transitioned to aircraft approved for flight-in-known-icing (FIKI), the opportunities increased as much as the flight planning. Additional systems needed to be checked and when I’m in the thick of it, the amount of monitoring substantially increases. In other words, there’s no free lunch.
Overlapping Systems
In this article, we’ll cover the basics of anti-icing systems, with another article to follow on de-icing. De-icing and anti-icing systems do overlap, like a Venn diagram. For example, a Cessna 402 has manually actuated boots and its AFM requires activation when ice accumulation reaches ¼- to ½-inch (traditionally a de-ice mindset). Meanwhile, the Pilatus PC-12 calls for turning on the automatically cycling boots prior to entering icing conditions (an anti-ice mindset). If your favorite ice removal system is not listed here, just wait for the de-icing sequel. Defining some terms might make this easier to understand.
Icing Conditions: This can vary depending on the manufacturer, but icing conditions can exist when the OAT (on the ground or for takeoff) or TAT (in-flight) is 10 degrees C or less and visible moisture is present.
Icing conditions also can exist without visible moisture while operating on ramps, taxiways or runways, where surface snow, ice, standing water or slush may be ingested by the engine, or freeze on the engine or the engine nacelle. Of course, icing conditions exist any time there is visible accretion.
Note that these definitions come from the manufacturer, not the FAA. The FAA’s definition comes from the so-called “Bell letter” (tinyurl.com/SAF-BellLtr), an interpretation produced by the FAA’s general counsel office in 2009: “‘Known icing conditions’ involve…circumstances where a reasonable pilot would expect a substantial likelihood of ice formation on the aircraft based upon all information available to that pilot.”
Visible Moisture: Clouds, fog or mist with visibility of one mile or less, rain, snow, sleet and ice crystals.
Anti-Ice: A procedure or system used to provide protection against the formation of frost or ice and accumulation of snow or slush on clean surfaces of the aircraft. Very effective when activated prior to ice accumulation, but not guaranteed to remove ice buildup.
De-ice: Systems designed to remove ice once accretion has begun. More on these systems in a follow-on article.
Aircraft Systems Affected
Icing conditions almost always mean IMC, or at best reduced visibility. The biggest threat to the accuracy of our instrumentation is a blockage of the pitot tube or static port, which ice can obviously do. One of the major reasons why we turn on pitot/static heat so early, and often in IMC regardless, is because water and fluctuating environmental conditions can cause water to freeze at unexpected indicated temperatures. First, even temporary instrumentation inaccuracy in IMC is an emergency. Second, and this point will be belabored quite a bit with anti-icing systems, it is much easier to prevent accumulation than to remove existing buildup.
Stall warning systems operate in a similar vein. If you have ever experienced a failure that caused continuous activation of the stall system, you know how disorienting and distracting it is. Conversely, if it is frozen in a position where it won’t activate, its valuable protection is lost. In every case I can find, electric heating elements are placed in the pitot/static ports and stall protection systems, activated by switches in the cockpit. It is important to preflight and operate these systems according to AFM guidance. For example, the FIKI-equipped Cirrus SR-22 requires the pitot heat to be on in visible moisture, and anytime the temperature falls below 41 degrees F. Fortunately, the SR22 will annunciate when this is required; not every aircraft is as capable. Keep the OAT/TAT in your scan, especially in the climb, as temperatures decrease.
Windshield
Ice creeping onto the windshield is never a good feeling. The only small benefit is that it is easier to track accumulation levels, which is sort of a mixed bag when that accumulation is blocking the view. I have seen three different types of windshield anti-ice systems. The first is heating elements integral to the windshield itself. Turning this on may heat all or a portion of the windshield, preventing ice formation. I flew a Piper Aztec with a similar system but external to the windshield. Lovingly referred to as hotplates, they provide heat on a portion of the windshield that allows enough line of sight to land.
The third method of clearing a windshield is TKS, further explained below. This system functions as a hybrid between anti-ice and de-ice. For reference, the Tecnam P212 AFM calls for windshield anti-ice as required and on demand. In light-to-moderate icing conditions, this means pressing the button every 5-10 minutes. The SR22 has a windshield defroster in addition to TKS fluid dispersal. Windshield defrost is required in icing conditions, and on the approach and landing checklist, the TKS push button is listed “as required.” I think of it like using windshield washer fluid.
Propeller
Propeller anti-ice is critical. Even a small amount of ice on a surface spinning as rapidly as a propeller can create a catastrophic imbalance. Additionally, if ice does build up and shed, it could strike the aircraft, especially in a twin. Similar to the windshield anti-ice, the two prominent methods of preventing ice accumulation is heat or TKS.
Propeller boots are heating elements located near the root of the propeller blades, where the relative speed is lower, and buildup is more likely. It is important to understand how the system in your aircraft works. For example, in the four-blade PC-12, the light propeller heat cycles 45 seconds on opposite blades followed by 90 seconds of rest. Heavy propeller heat runs on opposite blades at 90-second intervals with no rest. Obviously, the heavy cycle will help if there is concern about buildup beyond the capability of the light cycle, but if there is a degradation of electrical capability in icing, choices will need to be made.
Propeller anti-ice can be accomplished with a TKS system as well. A pump pushes fluid through a tube and into something called a “slinger ring,” located behind the propeller. Spinning at the same speed as the propeller, the centrifugal force moves fluid through the system and across the leading edge of the propeller. The fluid coating prevents ice accumulation.
An additional challenge across all propeller anti-ice systems is how to judge the prop’s condition. Use references like the windshield or wing boots in conjunction with how much TKS fluid is available and the estimated duration of flight in icing to make your choice.
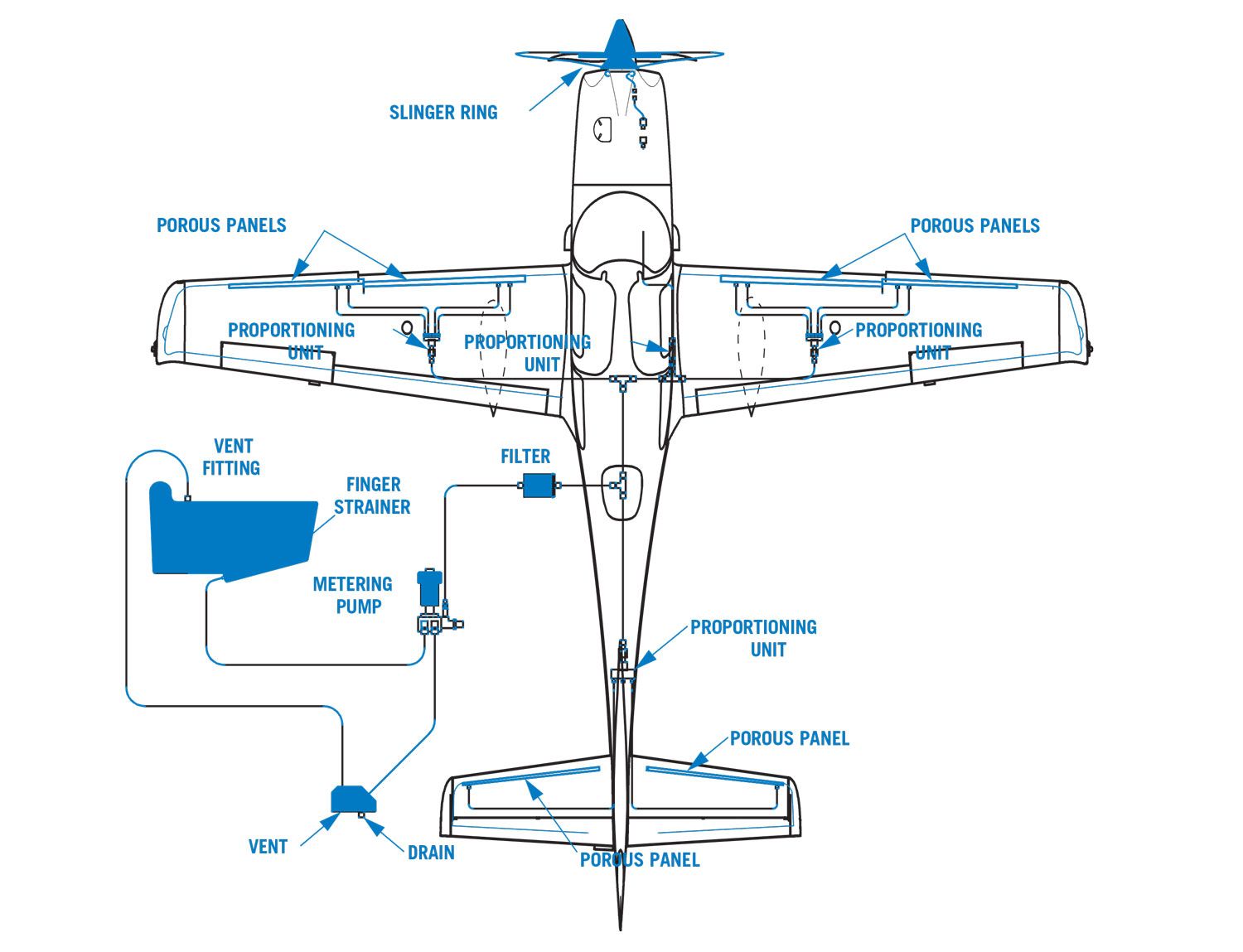
Referred to often in this article, TKS is one of the most popular forms of anti-ice protection, especially on general aviation aircraft. The acronym refers to Tecalemit, Kilfrost and Sheepbridge Stokes, the three companies that invented the process in 1942. Perhaps that little piece of trivia is so challenging to remember that many people refer to this system as a “weeping wing” because it slowly dispenses an ethylene glycol-based fluid. This lowers the freezing point of the liquid, preventing ice accumulation.
As shown above, the system consists of fluid reservoirs and pumps, plus leading-edge components with microperforations, allowing the fluid to flow onto flight surfaces. The propeller(s) and windshield also are “covered.” This system has very few moving parts, which increases reliability.
Additionally, the fluid tends to flow aft on the wings, prop and tail, providing ice protection on areas that are generally left unprotected by boots. Conversely, given the finite nature of the TKS system—the tank only holds so much fluid—a huge factor in operating in icing conditions is time. Ensuring adequate fluid levels exist (and then some) to outlast anticipated icing conditions is paramount.
Engine/Nacelle
Engine or nacelle heat is vital to avoid ice ingestion into the engine intakes. Generally found on turbine-powered aircraft, hot engine bleed air is used to prevent buildup on the protected surfaces. A small downside is the diversion of bleed air can decrease aircraft performance, which necessitates a friendly reminder that if you cannot maintain 500 fpm in the climb, ATC needs to know.
Another form of engine anti-ice may use engine exhaust to keep the intake warm. The PC-12 used this system, which worked great because it did not affect aircraft performance and there was no pilot input required. By far the biggest hazard was trying to put the intake cover in too soon after shutdown and melting it because the lip was still scalding.
Odd and Ends
Any system that calls for activation prior to entering icing conditions or designed with the intention of preventing ice accretion rather than removing existing building would probably fall under the envelope of anti-icing. Some planes have probes that work this way, and I found an iteration of a Cessna 402 with a heated fuel vent that is considered anti-ice. Best practice is to review AFM guidance and. if you are uncomfortable, consider alternatives or some winter weather instruction.
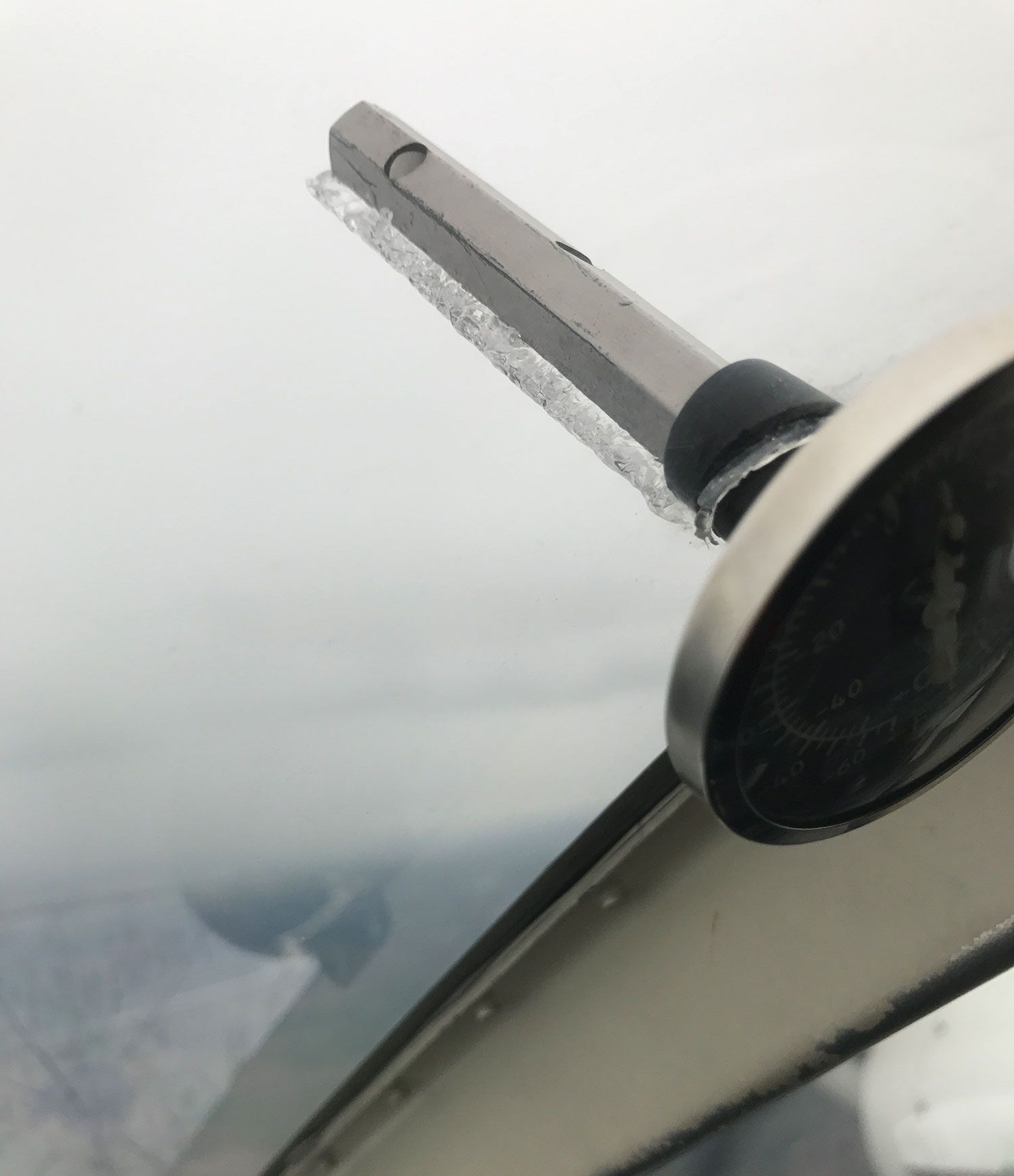
It should go without saying, but failure of a system that is required for operations into known icing while in icing conditions constitutes an emergency, and better weather needs to be found ASAP. Additionally, most FIKI-equipped GA planes are not designed to operate in icing conditions indefinitely. The systems are designed to keep the aircraft free of ice long enough to find more favorable weather.
If you find yourself in cruise, holding or otherwise continuously watching ice adhere to your aircraft, exercise your out. This could be any number of options, such as an altitude change, turning around or landing short of the destination. As with most things in aviation, flight into known icing is about good decision-making. Understanding your aircraft’s capabilities is a critical aspect of that decision-making.
Prior to enter icing conditions, I run through a flow. After completing the AFM-required actions for flight into known icing, I take a mental note of the aircraft state. Indicated airspeed, rough power setting and, if in a climb, a rough fpm value. I will also try to get a good look at the wing while it is clean. Between potential precipitation, reduced visibility and the inherent difficulty in seeing clear ice, accumulation will not always be obvious. Atmospheric conditions vary and this baseline cannot be relied upon without scrutiny, but it helps.
Even five knots of airspeed loss in cruise flight means the ice is winning and a different course of action must be considered. Many incidents and accidents occurred because pilots kept increasing power or otherwise compensated for the increased weight and loss of lift caused by ice accretion.
In a similar vein, it is good practice to disconnect automation periodically in icing conditions. Feeling how the aircraft handles can alert the pilot to excess trim or abnormal control forces caused by ice buildup.