We don’t usually take requests, but a reader wrote recently to ask us about tailplane stalls, those involving the horizontal portion of an airplane’s tail. It’s been a while since we covered them in-depth, so now’s a good time to revisit that topic. Our reader wrote: “The middle of summer when it is 90 degrees outside is not when most pilots think about tailplane icing but I would like to see an article about the aerodynamics of recovery from a tailplane stall and I know you need lead time to do that.
“The FAA guidance, as I understand it, is to pull back on the stick/yoke to recover from a tailplane stall. That seems wrong to me, but I have not been able to find an instructor who can explain the aerodynamics of how that recovery works…. I am sure the FAA is correct since they have people a lot smarter than me in aerodynamics. I am obviously missing something so could you please find someone who understands the aerodynamics of tailplane stall recovery and have them write an article on the subject in time for winter?”
Thanks for the story idea.
Upside Down
One of the keys to understanding tailplane stalls and the appropriate recovery method is to understand what the horizontal tail of a conventional airplane (i.e., one without a forward-mounted lifting surface known as a canard) does: It exists to counter the airplane’s natural tendency to nose down, thanks to its center of gravity being forward of the center of lift. It does so by exerting a lifting force opposite that of the wing. The diagram below may help.
Another thing that may help understand tailplane stalls is to refresh our memory on what a stall is. It’s when an airfoil—whether wing, tailplane or even propeller—exceeds its critical angle of attack (AoA) and stops producing lift. From our primary training, we recall that, for any airfoil, there is an angle of attack beyond which it no longer can generate lift. That’s called a stall. The cure for any stall is to decrease the airfoil’s AoA below that critical point, allowing it to again produce lift.
The good news is that in normal (i.e., non-icing) conditions, it’s hard to stall a tailplane. Even if you did, it likely would be momentary. The bad news is an ice-contaminated tailplane stall (ICTS)—the classic way for a tailplane to stall—may be difficult to recognize. And it doesn’t have to be winter for ice to form.
Tailplane Airfoil Conditions
Since a tailplane airfoil essentially is upside down when compared to a wing, pitching the aircraft’s nose up decreases the tailplane’s AoA, while pitching the nose down increases it. The diagrams below depict a tailplane airfoil in its normal and ice-contaminated states. It all looks bass-ackward until you realize the airfoil’s flat surface is on the top and the curved one on the bottom. Recovering from a tailplane stall requires pitching the nose up, not down.
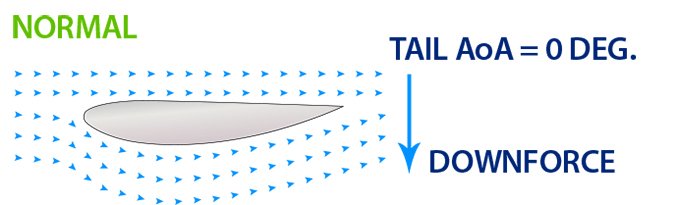



Recognizing ICTS
Most of us will never encounter an ICTS because we don’t fly in icing conditions. Yet icing can occur in all seasons, even over Florida in the summer, if you fly high enough. The punchline is if you think you may be experiencing an ICTS, you probably aren’t. That said, there are three basic telltale signs of an ICTS.
The first one may be lightening of the controls in the pitch axis, especially presenting as a nose-down tendency. Since pilots typically find it easier to push forward on a yoke or other pitch control, a pilot-induced oscillation may also be an indication of an ICTS. Needless to say, there may be difficulties trimming the airplane in such a situation.
Buffeting also may be a sign of an ICTS. This would occur as the tailplane airfoil “nibbles” at its critical AoA. This is the same sort of buffeting one would expect if the wing were at its critical AoA. However, it would be felt through the pitch control alone instead of through the airframe, as with a stalling wing.
A third sign of an ICTS may be the pitch control’s pronounced tendency to deflect itself forward, to the nose-down position. Some references suggest the pitch control may “pulse” or even suddenly be pulled aggressively forward, out of the pilot’s hands.
Recovering From ICTS
A likely occasion to encounter an ICTS is when deploying flaps in icing conditions. If the pitch control suddenly feels lighter than normal or wants to deflect to a nose-down position as you add flaps, retracting the flaps back to their previous position is advised. Also, reduce power, since higher power settings can force the tailplane to work harder. To answer the reader’s specific question, ICTS recovery is accomplished by pulling back on the pitch control, against all we’ve been trained to do in a stall. You may increase the wing’s AoA, but you’ll reduce the tailplane’s, which is key.
Regardless, it’s critically important to understand that stalling the airplane’s wing is much more likely to lead to losing control than is an ICTS, especially in icing conditions.
Sorting out if the wing or tailplane is stalling can be very tricky. Many in the aviation press castigated the pilot in the tragic Colgan Air Dash 8 -Q400 accident for fighting the stick pusher and pulling back on the yoke. It’s my belief he thought he was in a tailplane stall situation. If you remember there were NASA and FAA articles on tailplane icing / stall (especially in T tail aircraft) published before that accident occurred. Personally, I think it was a good research project that should have stayed as a technical paper.
“ICTS recovery is accomplished by pulling back on the pitch control, against all we’ve been trained to do in a stall. You may increase the wing’s AoA, but you’ll reduce the tailplane’s, which is key.” This still seems wrong. If the tailplane is already stalled, won’t pulling back on the pitch control increase the tailplane angle of attack and deepen the tailplane stall? In your diagrams, the “partially” and “fully stalled” figures represent increasing nose-up pitch control positions. The “Recovered” figure shows a typical nose-down pitch control position.
The article nods at this but it’s worth pointing out explicitly: as you get close to a tail stall you will lose pitch stability. The stability of an aircraft in pitch is due to the ‘decalage’ or ‘longitudinal dihedral’ of the wing and tail. This is a fancy way of saying the front-most lifting surface is set up at a higher angle of attack than the more rearward one. This disparity allows the plane to maintain pitch, and therefore speed, stability.
When the tail gets close to a stall it is also on the backside of the ‘power curve’ also known as in the ‘region of reverse command’. In this area near the stall airfoils start to generate less lift, instead of more, at higher angles of attack. Unfortunately this reverses the normal tendency of the tail to keep the plane trimmed for a constant pitch attitude and speed. As the plane starts to pitch down a tail in the region of reverse command will actually generate less force, not more. Without pitch stability it’s very hard to fly and it’s very easy to get the main wing into a stall without noticing.
This can all happen due to icing but the most common reason someone would lose stability is actually excessively aft cg. If you load the airplane outside of its cg envelope you are asking for more force out of the tail then it can provide, which means reverse command or a stall.
Zack, I should probably do a little more home work, but off the cuff, wouldn’t a forward CG cause the tailplane “to ask for more force” (higher coefficient of lift) than an aft CG situation? Airlines often work to have an aft CG loading to lessen the induced drag from the tailplane to improve fuel efficiency. Less induced drag = lower CL. I do agree that you lose stability with aft CG, but I don’t think I agree that an aft CG would cause a higher angle of attack (more force) from the tailplane. Am I wrong? Do I need to brush up on my Aerodynamics for Naval Aviators? Thanks.
The “Recovered” diagram is confusing because the relative wind is not coming across the tail horizontally making it very confusing when comparing to the other three diagrams.
Jerry – I had the same thought. When the tail stalls the nose is going to drop (since tail down force is lost) and you’re going to feel like you want to pull back on the stick, which is going to increase angle of attach (otherwise doing so wouldn’t have the tendency to pull the nose up!).
Zack, yeah, when slow, the tail will push down less, and the nose drops. This is what gives airplanes pitch stability. I don’t see this as an explanation of “you gotta pull” to recover from a tailplane stall.
Rather, it seems to me that a tailplane stall can only be fixed by decreasing the AOA of the tail = pushing. At the very least, this needs to be explained better.
I think this is a great article, but I think “tailplane” or “tailplane stall” is a subpar label. Seldom is ‘tailplane’ heard in the discussions of aerodynamics or flight controls. This is a “tail stall” and to me ICTS is an ice-contaminated TAIL stall. I’m not that smart when it comes to aeronautical terms, but if anyone can show me another reference where ‘tailplane’ is used, it would appreciate it so i can reference it myself.
I’ve never been satisfied with publicized explanations and every year about this time new articles ref back to initial writings on tailplane stalls…
My $.02 based on ancient aero degree and an aircraft that actually had a tailplane stall procedure for a different reason (unique interaction of oleo and flight control aerodynamic reactions on T&Gs)
I think the basic problem for those of us who don’t need engineers to talk slowly to explain that a tailplane is an upside down airfoil is how is pulling back reducing tailplane AOA? For an airplane sitting on its gear, pushing forward to unload tail would make sense, NOT pulling back more to increase tailplane AOA further. I think the subtlety that’s lost in published explanations is that for an aircraft flying with tailplane icing, the tailplane is still producing (less) downforce, not completely stalled. The confusion is that by pulling back you are not directly decreasing tailplane AOA by changing hinge angle, but you are inducing a nose up rotation of the airplane, this downward movement of the tail is what is reducing the tailplane’s AOA.
I’ve also seen a few articles talking about this being a real world factor only in flap deployment…and is the case in most problems, undoing what you just did (flaps back up) solves most of the issues.
Be nice to see new research summarizing real world experience since initial findings. Believe this whole discussion will eventually fall into the aviation and internet old wives tales that refuse to die, as applying much more narrowly than the Cassandras originally posited.
A Bartlett please respond to the comment several folks here have brought up. Pulling back on the yoke of a stalled horizontal stabilizer (previously providing down force) would increase the AOA of this “stalled” airfoil. How does this work?
The recovery still requires you to trade altitude for airspeed, then pull. I’ve experienced tail stall in iced-up 402, and float beaver. In my opinion, the main difference is the tail stall is more abrupt, and you’re likely to lose a lot more altitude. I felt the yoke go completely dead, as the DHC-2 pitched downward 30-40 degrees, much like an aggressive spin entry, but straight ahead.
I think some of the confusion in the comments stems from that the article and its images leave out the elevator, which when pulled back, changes the camber of the wing, just like flaps or ailerons do, increasing critical and effective AoA, coefficient of lift CL and (downward) lift (and of course drag) – as long as the icing hasn’t made that entirely impossible AND icing doesn’t affect elevator control!
Icing on the underside is probably worse than on top as the cambered/curved surface produces more lift than the flat(ter) one.
The images only depict a stabiliser /stabilator being moved, but this is not what happens in many GA aircraft anyway, and the yoke/stick always moves the elevator only (besides autotrim in some aircraft, e.g. Airbus), changing the camber and thus AoA and CL.
So, yes, when the stabilizer/stabilator stalls, the nose will pitch down and depending on the degree and location of icing hopefully un-stall but still producing less lift than normally in that position, and carefully(!) pulling back on the yoke will change the camber enough to increase the effective AoA (the relative wind onto the stabiliser/stabilator hasn’t changed in that moment) to produce more downward lift, bringing the nose back up.
Carefully, because the icing still has the (total) effective and critical AoA of the combination stabiliser/stabilator + elevator decreased and one can stall the tail again when pulling too much. The overall range of the elevator control will be decreased as long as there is ice and the range may be quite narrow but the only chance one has as that’s the only thing one can alter of the tailplane.
BTW, the tailplane pitch is actually what sets the (main) wing’s AoA, that’s why technically airspeed determines pitch and pitch determines speed.
See also av8n.com