Very few airplanes get precisely “book” performance. Some do better, some don’t quite meet the specs. Meanwhile, common piloting techniques differ from those recommended by the pilot’s operating handbook (POH) or airplane flight manual (AFM). It’s up to you to determine what is “normal” for your airplane, and the way you fly it. To learn what even a type-certificated airplane will truly do and when, you need to test-fly the airplane.
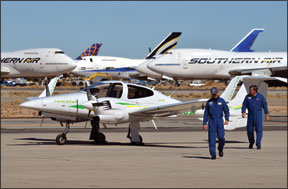
288
Pilot Technique
For a time, I was the lead instructor for Beechcraft Bonanza training for an international flight safety organization working at the Beech factory airport. Part of the training we provided included flight in the customer’s airplane.
One of the items on the flight training syllabus was to show different flying techniques, and compare actual performance received to that predicted by the POH.
For example, the POH for this particular make and model of airplane calls for applying full power for takeoff prior to brake release, then accelerating in a low angle of attack to a specific liftoff (sometimes called “rotation”) speed and pitching positively upward to a VX attitude. To get the POH performance, you must use the POH technique.
Except when taking off from a truly short runway with wires or a tree line just off the departure end, almost nobody actually takes off like that. Instead, we tend to feed the power in gradually, allowing the airplane to roll as power comes in, ensuring adequate cooling and minimizing prop erosion. As the aircraft accelerates, we add nose-up pitch to reduce the weight on the nose wheel, and to put the airplane into an attitude from which it will smoothly lift off “when it’s ready to fly.” Most of us then bring the nose slightly upward to permit the aircraft to accelerate in something closer to a VY attitude. This technique is smoother and more airplane- and passenger-friendly than the “book” takeoff technique. But guess what: You won’t get the book takeoff performance doing it this way either.
To see how a particular airplane I commonly fly (a 1970 A36 Bonanza with an IO-520 engine and three-blade propeller) actually performs, I did five test flights taking off using the smoother, passenger-friendly rolling takeoff and lift off “when it’s ready” technique. I conducted my tests at roughly 200 pounds below the airplane’s maximum gross weight, in as close to zero wind and zero crosswind as I could find in central Kansas. Starting each takeoff test run with the nose wheel at the very beginning of the first runway stripe, and knowing that the standard runway-stripe-plus-space distance is 200 feet, I could estimate the distance required to lift off using this non-POH technique. I set the altimeter on zero for these tests and, using a runway stripe count, also estimated the distance traveled upon reaching 50 feet above runway height. I averaged the estimates for ground roll and 50-foot distance actually observed in the five tests, and compared those to the figures calculated using the POH’s takeoff performance chart.
I found my results were consistent. The average takeoff ground roll distance was about 45 percent longer than the book figure under the same conditions of temperature, field elevation and airplane weight. The average distance required to attain 50 feet of altitude was 50 percent greater than book using this common piloting technique.
The POH does not include a performance chart for taking off with partial flaps. I did not test departures using roughly half flaps (15 deg.), but could have done so and derived a second correction value to the POH takeoff chart if I wished.
Yes, I could probably have derived more precise data some other way, including having a second pilot aboard to do the runway stripe-counting (this would have been especially true for the 50-foot distance estimates). What I found, however, is that, at least on a warm day (70 deg. F) in central Kansas (at roughly 1500 feet msl), adding 50 percent to the calculated POH takeoff distances before adding any additional margin for safety seems to be a good estimate of the type of performance I routinely get in this A36.
The experience also confirmed that if I am taking off from a truly short runway or to clear obstacles, I need to use the “book” takeoff technique from the POH.
Wide Variation
In another job, I learned there is a wide variation in performance from one individual airplane to the next. I was a production test pilot for an STC firm that added aftermarket turbochargers (“turbonormalizers”) to Beech Bonanzas, Cessna Cardinal RGs and even Cessna 185s. The company’s sales literature said the Bonanza modification resulted in a 200-knot airplane. And most of the time it did. Every now and then, however, we’d deliver a 199-knot Bonanza…and the owner was disappointed that it was only 30 knots faster than “book.”
I began doing a pre-modification test flight to collect data to compare to post-modification tests. I did this to show the owners the actual effect of modifying their airplane—the benefits side of the cost/benefits decision. What I found, however, was somewhat surprising to me: Before modification, I found as much as a 10- to even 15-knot difference in maximum true airspeed between outwardly identical Beechcraft Bonanzas. In concert with our engineers, I learned several factors could affect the actual TAS received:
Airplane rigging. A minute change in wing or tail angle of incidence (the angle at which it is mounted relative to the airplane’s longitudinal axis) can make a significant change in drag and therefore TAS. In fact, there’s a cottage industry in “tweaking” some airplane types for maximum speed, and in many cases this involves adjusting the wing’s angle of incidence.
Engine power output. Some engines are just healthier and more powerful than others. Cylinder compressions, ignition timing and the quality of the wiring harness and spark plugs can all affect power output. Tachometers frequently are found to be slightly out of calibration, but off enough that a pilot is developing less (or more) power than the indicated RPM suggests.
Center of gravity location. An aft-loaded airplane will fly faster than one with a more forward center of gravity, all else being equal.
Armed with pre-modification results for individual airplanes, I did find an interesting pattern. In pre-mod data gathering flights, I used full throttle, 2500 rpm and a “maximum horsepower” mixture setting (75 deg. F rich of peak exhaust gas temperature, or EGT—this was before the repopularization of lean of peak cruise operation). I conducted the speed data run at 10,000 feet msl, in part because the field elevation where I worked was 7600 feet, making lower altitude tests challenging. After the turbo mod was added, the same airplane would average about 10 percent faster at 10,000 feet, using 25 inches/2500 rpm and a maximum horsepower mixture. At 20,000 feet, the highest altitude to which we tested the modification at the time, the airplane would average 18 percent faster than the pre-mod 10,000 feet speed.
These percentages held consistently true across the entire fleet of airplanes we modified—the Bonanza, the Cardinal RG, the A185 and even a never-certified Aero Commander 112 we tested. A fast airplane coming in for modification left a very fast airplane. A slower airplane in pre-modification tests came out a slower, but still very fast airplane.
With or without an engine modification, the only way to tell for certain how the airplane will actually perform is to do some individual airplane flight testing.
Engine Swap
Here’s a very common question I’m asked: I own a Beechcraft F33A Bonanza and have upgraded from its original, 285-hp IO-520 engine to a 300-hp IO-550. The STC [Supplemental Type Certificate] supplement does not include any performance information. How can I predict the performance of my modified airplane for flight planning and for input into my flight planning software?
In most cases, STC holders are not required to provide updated performance data for airplanes modified with their product. When granting approval to the modification the FAA usually accepts a statement that the airplane “meets or exceeds” its original performance as sufficient for flight planning purposes—assuming the pilot will continue to use the aircraft’s original POH or AFM performance charts, and the added horsepower will result in a greater margin of safety than originally certified for the design. While this is certainly true for takeoff and climb performance, and may be true for cruise speed (where drag is as much a factor as increased horsepower), you can make the case that this isn’t true for fuel burn and endurance figures (more power generally means more fuel burn). And more horsepower will have no impact on landing distance, when power is essentially zero. Finally, it certainly isn’t true for other modifications, especially those increasing an airplane’s gross weight.
Part of the fun of modifying an airplane, I’ll often tell pilots who ask this type of question, is finding out just what your airplane will do with the modification that’s been added. To do so, you need to flight test your airplane.
Leaning Techniques
Most airplane handbooks include tables of expected cruise performance speeds at a range of power settings. Frequently, especially in older airplanes, the tables are based on a specific percentage of power. In some POHs, the tables are purely a function of propeller speed, and do not correlate to a specific percentage of power. All assume a consistent leaning technique—usually 25 deg. F rich of peak EGT, although some airplanes (the 1984 and later A36 Bonanza, for example), include tables for both 20 deg. C rich of peak (ROP) and 20 deg. C lean of peak (LOP) EGT.
The actual cruise speed and fuel flow may differ from tabulated predictions. Further, if you use a leaning technique differing from that assumed by the POH charts, then you will not get POH cruise speeds or fuel burn. For example, if you fly your airplane LOP where no LOP data appears in your handbook, or if you fly 75 deg. F ROP for additional cylinder cooling when your POH data are based on 25 deg. ROP. These differences will directly impact your airplane’s range and endurance. How can you accurately predict your airplane’s cruise performance? You guessed it—by doing some flight testing using the techniques you commonly use.
Vortex Generators
Aftermarket vortex generators (VGs) are a popular modification, especially on twin-engine airplanes. A VG installation alters the airflow across the top of the wing (and in some cases, on the tail as well). Most VGs are designed to enhance laminar flow, that is, to keep the airflow “attached” to the wing surface for a greater distance aft along the wing’s chord line, especially at high angles of attack. This means there’s more lift for a given angle of attack, and the generation of the same amount of lift at a slower airspeed than before adding VGs.
Vortex generators are often touted in multiengine circles as reducing the airplane’s VMC and enhancing single-engine climb performance. Because lift is preserved at slower speeds, VGs also permit shorter takeoffs. Since landing ground roll distance is a function of the airplane’s inertia and therefore the speed of touchdown, the slower final approach speeds permitted by adding VGs result in dramatically reduced landing distances.

That last criterion, landing distance, was the reason a company I worked for added VGs to a 58TC Baron we operated. The 58TC is much heavier than most Barons, and is not a short-field airplane. I conducted pre- and post-modification flight tests on the 58TC and found it indeed stalled at a slightly slower airspeed. More importantly, VGs noticeably improved aileron authority at final approach speeds, making it much more comfortable to slow to the book or VG-lowered final approach speed, and thereby land in much less distance than before the mod.
But there’s never a free lunch, and this mod came with two downsides: First, there was no data at all in the VG STC’s supplement to determine the new approach speed or landing distance. Second, at least in Beech Barons (and verified by a U.S. Forest Service-commissioned study by the University of Tennessee Space Institute in the early 1990s), VGs virtually remove all pre-stall buffet or warning—the wing is flying fine until it stalls with no warning. So how did I arrive at a new final approach speed and estimates of short-field landing distance? Only by test flights of our type certificated (and supplementally altered) aircraft.
AOA
Angle of attack indicators are the latest craze in light airplane aerodynamics. The basic AoA indicator has been with us for many, many years, and are the standard takeoff, climb, approach and landing reference in most military airplanes and many corporate jets. They are only now receiving a lot of press for installation in light, piston-powered airplanes as a possible tool for reducing the stall-related fatal accident rate.
An AoA installation is not an exact science, however. They don’t come aligned for a specific airplane’s VX, VY and VREF approach indications and must be calibrated after installation. This means a pilot must take the newly AoA-equipped airplane up and very precisely fly the target airspeeds, then adjust the AoA sensor to correspond to the tested speeds. There is wide latitude, also, as to exactly how an individual pilot sets his or her AoA device to indicate. What’s this all mean? If you wish to add an AoA display to your airplane, you (or someone) has to very precisely test-fly your airplane.
Gross Weight Increases
Who wouldn’t want their airplane to carry more weight? There are many STCs available to increase the maximum gross weight of a large number of airplanes. Like engine modifications, however, there is no requirement for an STC holder to provide revised performance data for flight at the higher weights. This is counterintuitive because a heavier airplane will naturally perform less well for a given maximum horsepower. Increased weight increases stall speed, and therefore takeoff and landing speeds—degrading takeoff and landing distance performance. Although a few gross-weight-increase STCs give some general rules of thumb for adjusting performance calculations, in all cases it’ll take some individual-aircraft flight testing to determine the actual effect of flight at higher aircraft weights.
Why Flight Test? No airplane will perform precisely to “book” performance. Modify the airplane and/or your piloting technique from the original assumptions under which POH performance expectations were derived, and actually performance will differ from expected even more. The only way to predict the performance of your airplane, flown the way you fly it, is to conduct your own flight tests under controlled conditions and then to document the results. After all, learning what your airplane will actually do is part of the fun of owning and flying it.
Tom Turner is a CFII-MEI who frequently writes and lectures on aviation safety.