
While visiting France a few years ago, I found an Aero Club that would let me fly with one of their pilots. At the club I was introduced to M. Bus, a retired businessman. My French was barely better than his English, but we spoke the common language of aviation. The airplane was a Piper Archer, examples of which I’ve flown all over the U.S., and the preflight went smoothly until we checked the fuel level. You’re probably familiar with the small metal “tab” in many fuel tanks indicating a partial fuel level. This Archer’s fuel level was well below the tabs.
“That’s not enough fuel,” I said. In French, “fuel” is “essence.”
“I agree,” replied M. Bus.
But the fueler was already closed for the day. Luckily another club Archer taxied up, was available and had plenty of fuel, and we took it for a lovely flight along the Mediterranean coast. That experience led me to think of the kinds of energy we use in flying as “essence.”
Fuel is chemical energy, but it’s not the only kind of energy that keeps an airplane flying. Essence is a more useful concept than energy: While energy can neither be created nor destroyed, some forms of essence in flying can vanish. When they disappear, the consequences can be disastrous.
My use of “essence” describes most things that move: dynamics. To make it aerodynamics, use another concept, “finesse,” which is what the French call glide ratio. Some engineers call this efficiency. Finesse is a more useful way to say glide ratio because its English meaning also includes how many of us try to fly.
THE FOUR FORCES
Primary flight training introduces the four forces of flight: lift, weight, drag and thrust. As a refresher, weight is the effect of gravity, which basically points down to the Earth’s center. The pilot has some control over weight by deciding how much stuff to put into the airplane. Burning fuel sends some weight overboard through the exhaust, which can be a problem for lighter-than-air craft, as described in the sidebar on the opposite page.
Lift is created by manipulating the airflow, mostly using the wing. It acts perpendicular to the relative wind, so it has a rearward component in a climb and a forward component in a descent. Drag acts parallel to but opposite the relative wind. Thrust acts parallel to the relative wind, but in a more desirable direction.
THE ADVANTAGE OF ESSENCE
The four forces have to balance to keep the aircraft in a steady state. In level flight, this means that thrust equals drag and lift equals weight, which is depicted in Figure 1 on page 19. Consider a zoom climb in, say, a Cessna 172. Start in level cruise at 100 KIAS with the flaps up. Clear the area. Now, pull back on the yoke to raise the nose around 15 degrees. Altitude increases and airspeed decreases, what airshow announcers might call “trading airspeed for altitude.” A steady-state climb is depicted as Figure 2 on page 19; the zoom climb is more complicated. Lower the nose as the airspeed nears 50 KIAS and you will be a few hundred feet higher than you started.
After the climb, the engine’s thrust will increase the airspeed from 50 KIAS back to 100, apparently an increase in energy. Where did this energy come from? The engine converted the “essence” stored in the fuel tanks into thrust (as well as heat and noise). Once back to a steady state, the zoom climb doesn’t change the energy of the aircraft much at all—kinetic energy in the form of airspeed was converted to potential energy in the form of altitude. This is the nature of what I call the essence of flying.
But as a pilot maneuvers an aircraft, interactions between the four forces become complicated. What does it mean to say that we maneuver aircraft even when we’re not maneuvering? Any maneuver converts energy from one form to another, so even straight-and-level flight becomes a maneuver because the airplane is converting chemical energy in the form of fuel into (mostly) thrust.
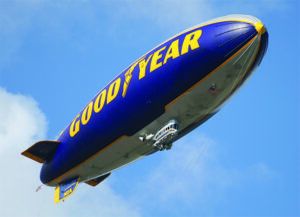
One way to clarify aerodynamic concepts is to apply them to airships, gliders and helicopters. Take lift, for example, and consider that it’s possible to have too much lift. How can that be true?
Airship lift comes from a lighter-than-air gas, originally hydrogen but today helium is the norm. The gas is contained in rubberized bags (which helps explain why Goodyear, a rubber company, is in the airship business). The gas is buoyant so it rises, carrying the ship with it, until reaching equilibrium with atmospheric pressure. The volume of the gas determines how much weight it can lift: A liter of helium can lift about a gram.
The gas bags don’t fill the hull of a non-rigid airship; air bags called ballonets help preserve the shape. They are full at takeoff and expand as the ship rises. If internal pressure becomes too high, the crew can release air from the ballonets to maintain shape and trim. Once the ballonets are empty, trim is maintained by venting expensive helium overboard. In this condition, the ship has too much lift.
As an airship travels, its engines burn fuel, just like an airplane. After only a few hours, an airship will be significantly lighter, and its lifting gas will provide too much lift.
PILOT ESSENCE
To me, “essence” is the total of all of the energy available in the aircraft. But there’s more to energy than fuel and height and speed. The flight begins with the aircraft sitting still. The engine starts, burning fuel, at first changing it into noise and heat. It begins to move, and approaches the runway, burning more fuel, now not wasted. Burning even more fuel the aircraft gains speed, then height. Speed is a form of energy, and with enough speed the craft can fly, gaining height, a different form of energy. Climbing at constant speed means the “speed” part of essence doesn’t change. Climbing increases height, the potential part of essence. The combination consumes the chemical part.
Energy cannot be destroyed, so height becomes speed and speed becomes height, as the pilot wishes. The essence of fuel has become the essence of the craft, energy describing its dynamics. The pilot guides this transformation. The throttle is set, the elevators are moved, the flaps and wheels go up and down. The pilot’s hands and feet move, and that movement is also part of the essence of flight.
It is fueled by the food we consume, the Sun’s essence becoming, through plants, an energy store—sugar, perhaps—becoming kinetic energy in the form of muscle movement. This sugar also fuels the pilot’s brain. Experts say that our brains account for about 20 percent of the body’s energy consumption. Using the marketing standard of 2000 calories per day, that’s about 400 calories worth of energy, about the contents of the candy bar whose advertisements take a humorous view of how stupid we can become when blood sugar drops.
Running out of “brain” essence is as bad as running out of fuel. Recently, the instrument-rated owner of a high-performance single told me about a Friday evening departure for a 600-nm trip. He works hard in information technology and was tired. He decided to wait until the next morning. While he didn’t say it this way, he did not have enough mental essence to make the trip safely.
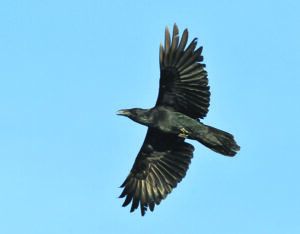
While hiking in the desert, you see a raven take off and watch it fly directly overhead. You watch its wings flap and you hear a distinct whoosh with each flap. That is the sound of lift, and of thrust. The raven converts chemical energy (sugar) into muscular energy, which in turn becomes flight.
I suspect many pilots have wondered what it feels like to fly like a bird. Richard Bach speculated about this in Jonathan Livingston Seagull, making it sound like maneuvering can be painful. That seems right to me: I imagine that each flap of a bird’s wing feels something like doing a pushup. Twenty of either seems like a lot.
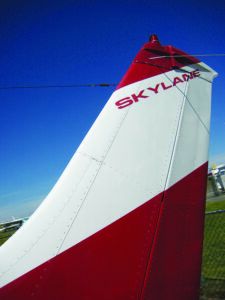
Most small planes are noisy enough, so sometimes it’s hard to hear the sounds it makes. It’s easier in gliders, where pulling on the speed brakes causes a distinct rumble. Cessna 172s and 182s seem to have more rumble at full flaps than Cherokees and Mooneys, while many Cessna singles also have a distinct rudder rumble in uncoordinated flight.
Noise like that is moving air, which has energy simply because it is moving. That noise is the sound of energy being dissipated.
DECLINING UTILITY
As the flight goes on, it becomes more difficult to transform essence. Neither speed nor height can be transformed into fuel. As the craft descends, its height becomes heat, and heat is almost always waste. And the fuel continues to disappear. If there is a crew of two or more, essence is used discussing the potential plight of using too much fuel, while a single pilot must wrestle alone with the question. The tank is too soon empty.
Or the pilot can run out of ideas. As the flight goes on, the pilot becomes tired, or hungry or thirsty. The tank is too soon empty. Or the pilot can lose focus to distraction. Something trivial but broken—heat, cold, electronics, politics, other distractions. All steal essence from the pilot. Again the tank is too soon empty.
ENERGY CRISIS
After Fred Noonan’s navigating skills and who gets the left seat, it’s the oldest debate in aviation: Does the elevator control airspeed or does it control altitude? Does power control altitude or does it control airspeed? Both students and flight instructors struggle with the pitch/power problem. The student asks, and the instructor hems and haws and says things that start with “Well, sometimes…” or draws out a long list of conditionals (“If you’re high and the airplane is slow and it’s a Tuesday in the Northern Hemisphere, then you should…”). Is there a simple answer? Yes. The answer is essence.
But let’s back up a little bit to a problem where the relationship between pitch and power is clear. The instructor asks how to recover from a stall. The student is confused, because the publications use flowery words about detached flow and burbling air to talk about why an airplane stalls, rather than saying what a stall is. A simpler definition of stall cues the recovery: A stall means that the angle of attack is too high. So to recover, the pilot must reduce the angle of attack.
Students whose instructors fail to emphasize this concept have a dangerous attitude about stall recovery, because they do not have a clear idea that it’s pitch, not power, that will save the day. Too often you hear this dialog:
Instructor: “How do you recover from a stall?”
Student: “Add power and…”
Instructor, interrupting: “What do we do in gliders?”
Adding power means changing essence; stall recovery means reducing the angle of attack. Power pulls the pilot away from the ground. (Admittedly, power slightly reduces the stall speed of many light aircraft, so it may in fact aid stall recovery, but that is a secondary effect.)
Now return to the original debate about pitch and power. The solution to the conundrum is to examine the nature of power. Power becomes one of the four forces, namely thrust, although it also involves a small amount of lift at higher deck angles. A force means that there is a change in energy. Energy is essence. Essence is the key.
Two parts of essence come to the fore: potential energy, growing with altitude, and kinetic energy, growing with speed. Essence is conserved, so losing one increases the other. “Trading airspeed for altitude” is how gliders go around during a balked landing (a dangerous maneuver that I do not recommend).
But essence changes. The only way to change it is to exert a force. Two of the four forces are important here. Drag tends to reduce useful energy: it slows the aircraft, transforming essence into friction and heat. That’s one reason gliders are so small and sleek. The reduced drag means that the glider retains more of its energy. How reduced is the drag? A Cessna 172’s lift-over-drag ratio is about 9:1. Many gliders boast a lift-over-drag ratio of 39:1. At the same weight, the glider has ¼ of the drag! The other force is thrust, which increases dynamic energy.
And that’s the debate: does adding thrust force the craft to speed up, or does it force the craft to go up? It’s both. Thrust adds dynamic energy, which can be in the form of speed (kinetic energy) or altitude (potential energy).
Gliders don’t have thrust, and depend on rising air to gain potential energy in the form of height. Rising air is moving air, so that’s kinetic energy. Airplanes can gain a little energy this way, but usually depend on stored chemical energy, the first form of essence.
But you knew this already: “An airplane climbs because of excess thrust.” A climb increases the airplane’s potential energy, at the cost of chemical energy.
WHICH IS IT?
So, there is a definitive answer to the question of managing power during approaches: If the essence (energy) is low, add power; if the essence (energy) is high, reduce power.
In practical terms: Add power when low and slow; both forms of essence are needed. Reduce power if the PAPI is all white; you are high, so you have too much energy. These two change how essence is converted.
Leave the power alone if you are low and fast. Raise the nose to convert airspeed into altitude (just don’t let the speed get too low). Leave the power alone if you are high and slow. Lower the nose to gain speed, and you will lose altitude.
And have a snack before you start the approach.
Jim Wolper is an airline transport pilot and mathematics professor. He’s also a CFI with single-engine, multi-engine, instrument and glider ratings.