Long ago, an instructor explained to me that knowing the various options for using the airplane, the different ways to make it do what’s needed, and the savvy to use those different models as appropriate, differentiated aviating from rote piloting. In the case of using climb abilities to your benefit, the best preparation begins with knowing and understanding all available options, knowing the plane and practice.
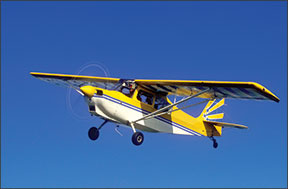
288
How we chose to perform our climbs should be tailored specifically to the time of day, the weather, the surrounding terrain and/or the traffic. We can chose to maximize the trip’s average groundspeed, minimize fuel use or get as high as we can as fast as we can, fuel efficiency be damned.
Born of Imbalance
You, of course, remember the four forces acting on all aircraft in flight: thrust, its counterpart drag, lift and its polar opposite, gravity. In stable, level flight, the two force pairs cancel out each other; thrust and drag balance shown by the aircraft holding a steady speed; and lift balances gravity as evidenced by the aircraft’s constant altitude. Alter that balance between any one of the four and other things also will change as the balance of forces is destabilized.
Climb results from lift exceeding gravity—a change of state produced by the use of power in excess of that needed to sustain stable, level flight at any given pitch setting.
At any given airspeed, any increase in applied power initially accelerates the airplane as it seeks to return to its previous trim attitude—and thus its previous airspeed. That excess speed first turns into altitude gained on an initial climb until the airplane, left alone, stabilizes itself at the old airspeed—but at a steady climb proportional to and consistent with the amount of power added.
Similarly, an aircraft at cruise gradually trimmed to a lower airspeed will, leaving all else alone, gradually begin to climb because the power used exceeds that needed to sustain level flight at the lower airspeed. But that’s not all that’s going on in a climb.
According to the FAA’s Airplane Flying Handbook (AFH), FAA-H-8083-3A, “In a climb, weight no longer acts in a direction perpendicular to the flightpath. It acts in a rearward direction. This causes an increase in total drag requiring an increase in thrust (power) to balance the forces. An airplane can only sustain a climb angle when there is sufficient thrust to offset increased drag; therefore, climb is limited by the thrust available.” In other words, we must have sufficient power, in excess of that required for straight and level flight, before we can climb.
So it stands, the more power we have in excess of that needed for level flight, the greater the climb rate—at least, as long as the engine makes power enough to sustain that climb.
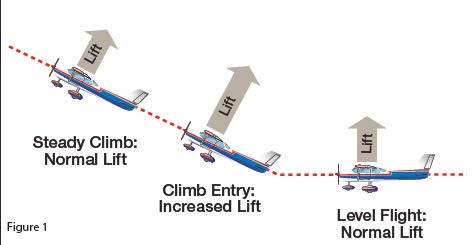
288
At some point, the change in altitude results in reduced power or an arrival of indicated airspeed at the stall point on the airspeed indicator—and all rules are off. If the aircraft reaches a point at which it can fly but not climb, it’s at its absolute ceiling. At that point and all things begin equal, the aircraft is unstable, bordering on uncontrolled. But at least you’ve plenty of air beneath you, giving you time to correct your situation and restore your control over the aircraft.
The Forces in Climbs
For all practical purposes, the wing’s lift in a normal, steady-state climb is the same as it is in a steady level flight at the same airspeed. Although the aircraft’s flight path changed when the climb was established, the wing’s angle of attack (AoA) with respect to the inclined flight path reverts to practically the same values, as does the lift.
Initially, there is a momentary lift increase: “Raising the aircraft’s nose increases the AOA and momentarily increases the lift. Lift at this moment is now greater than weight and starts the aircraft climbing. After the flightpath is stabilized on the upward incline, the AOA and lift again revert to about the level flight values,” according to the FAA’s Pilot’s Handbook of Aeronautical Knowledge (PHAK), FAA-H-8083-25A. This is depicted in Figure 1, above.
Rate or angle?
Most pilots’ formal climb training involves some short-field work, perhaps along with exercises focused on maintaining a specific attitude or airspeed. When training to become a multi-engine pilot, we spend a lot of time seemingly trying to balance a large, underpowered airplane on the head of a pin, where any failure to maintain the correct pitch and bank angles is immediately noticed on the vertical speed indicator.
Other than that, we’re usually taught there are two speeds to fly when climbing, one for a best-rate climb and another for the best angle. The best rate of climb speed, VY, is the one delivering the maximum gain in altitude per unit of time. It’s usually higher than the best angle of climb speed, VX, which provides the greatest altitude gain over a given distance. It’s the one you aimed for when doing those aforementioned short-field exercises. A third speed, VYSE, best single-engine rate of climb, is published for multi-engine airplanes. Interestingly, it’s also the only best-climb speed marked on an airspeed indicator.
Reams have been written about which kind of climb is best. Best-rate climbs may get you to altitude quickest, but you’ll likely see the lowest average airspeed across the route. If ATC says “expedite climb” to your final altitude, there’s no question: Use VY. If your departure route takes you toward terrain higher than your departure point, consider VX. More important is remembering to determine the distance to the obstacle and whether climbing at any speed will keep you clear of it.

288
The other thing to keep in mind about VY and VX is they’re published for an airplane at sea level and change as we climb. Since we very rarely depart a sea-level airport, and since the act of climbing means we’re rather quickly higher than zero feet, we need to adjust our best-climb speeds as altitude increases. The chart on the previous page, Figure 2, details how these speeds change with altitude—VY decreases while VX increases, slightly—all the way to either the airplane’s service ceiling or its absolute altitude.
Cruise Climbs
After best-rate and best-angle climbs, a third type also is worthy of discussion: the cruise climb. In general, cruise climbs raise the average speed for the trip, a bit, and lower overall fuel use—particularly when the pilot practices progressive leaning. Adjusting fuel flow downward every turn of the thousand-foot needle of the altimeter saves gas and comes closer to optimizing power. If balancing groundspeed against rate of climb is what you need, a cruise climb is the way to go.
Cruise climbs, of course, lack a definitive V-speed, which makes some sense: It’s a lot more variable than VX or VX—despite the need to modify these two speeds for altitude—and depends more on operational requirements and individual choice than anything else.
A cruise climb is one conducted at an airspeed higher than the best-rate or best-angle climb speeds and produces a lower climb-rate number used to trade off gain for cross-country progress. Cruise climbs not only speed you more quickly toward your destination, they also save fuel, help the engine run cooler and improve the view from most airplanes. In fact, it wouldn’t be hyperbolic to say that most airplanes gain altitude in a cruise-climb configuration rather than one adhering to published speeds. More on cruise climbs in a moment.
Setting up for climbs
Every climb requires proper airplane configuration. Factors to consider, in addition to obstacles or climb rate, include engine cooling, out-the-cockpit visibility, minimizing drag, setting power and weather.
For maximum-performance climbs, that means open cowl flaps, if so equipped. With cooling-air flows reduced by the higher angles and lower airspeeds at VY and VX, the cowl flaps will be needed to increase the flow of cooling air coming through the cylinder fins and oil cooler. If opening the cowl flaps isn’t enough, or your airplane doesn’t have that option, excess fuel can be used to keep things cool under the cowling. Enrichening the mixture to, say, 150-200 deg. F rich of peak EGT and consistent with altitude has little-to-no effect on a piston engine’s power production.
Unless needed to sustain a special rate or angle, flaps and gear should be up as quickly as practicable. If speed, however, underpins your climb decision, remember to close those cowl flaps as soon as safe—shortly after trimming to your cruise-climb setting.
And that trim knob, crank or wheel, is seldom more your friend than when setting up the aircraft for a sustained climb. How better to assure a stable, consistent ride up the atmospheric stepladder than by trimming the aircraft for the exact speed you need to hit for cruise climb, obstacle climb or best-rate climb.
When normal won’t work
Some call it a “zoom” climb—others may have their own name—but this type of climb results from letting speed build and then hauling back on the pitch control to convert velocity into altitude. For some airport and aircraft combinations, a zoom climb may be the only way out.
This is a recommendation to think seriously about the necessity of the flight or the wisdom of the plan if you have to factor into your departure plans for anything like a zoom climb.
Abnormal climbs may also be appropriate for departing a short field in soft conditions—or a short field, period. Don’t let a focus on minimizing takeoff distance and maximizing immediate climb become the enemy of remembering to maintain a comfortable margin above stall speed—in particular if the winds are at all variable.
It’s no fun being close to the ground, at a high pitch angle and higher AOA—only to have strong winds suddenly subside or, worse, reverse direction. And low-level stalls hurt the worst.
Meanwhile, anything that adds drag to an airplane will handicap its performance. A climbing turn, despite how necessary it may be under some circumstances, qualifies.
Absent some reserve power, putting your climbing airplane into a turn will diminish the climb’s rate and, possibly, its angle. If you’re trying to climb out of a box canyon or skyscraper-studded neighborhood, you need more leeway if a turn has to be part of the departure.
Planning Your Climb
Consider this scenario: 500 nm to go and the need to fly at 7500 feet msl after taking off from sea level. What’s the most efficient way to get to your cruising altitude?
My long-time traveling machine boasted a full-throttle, best-rate climb of about 950 fpm at 82 KCAS at sea level. Its best-angle climb produced 750 fpm at 65 KCAS. Using those numbers, which admittedly are based on sea-level performance, means I’ll need just under eight minutes to reach my target altitude at maximum-rate climb. In that time, I’ll cover 12 nm. If cruising at 135 knots groundspeed for the rest of the trip, I’d need 3.65 hours for a total of 3.75 hours—three hours, 45 minutes—to arrive at my destination.
If I chose the airplane’s best-angle climb speed, I’ll need 10 minutes to climb to 7500. I’d cover the same 12 miles or so, but spend two more minutes doing it. All else being equal, I’ll spend about three minutes longer en route when climbing at best angle instead of best rate.
But if I used a faster speed after clearing any obstacles, the numbers change again. Trimmed to 115 KIAS for the climb, experience tells me a 500-fpm rate was available. Yes, it took longer to get to 7500 feet, but I would be at nearly 29 nm from my departure point, or more than twice the distance toward my destination.
My total time en route is about 3.4 hours, meaning I can arrive about seven minutes earlier and burn three to four fewer gallons of fuel than the other two options.
Once clear of obstacles and with enough air underneath to lengthen the time available for emergencies, climbing at a faster airspeed pays off for me. It will for you, too.